Rechargeable metal-air batteries as a cost-effective alternative to lithium-ion batteries
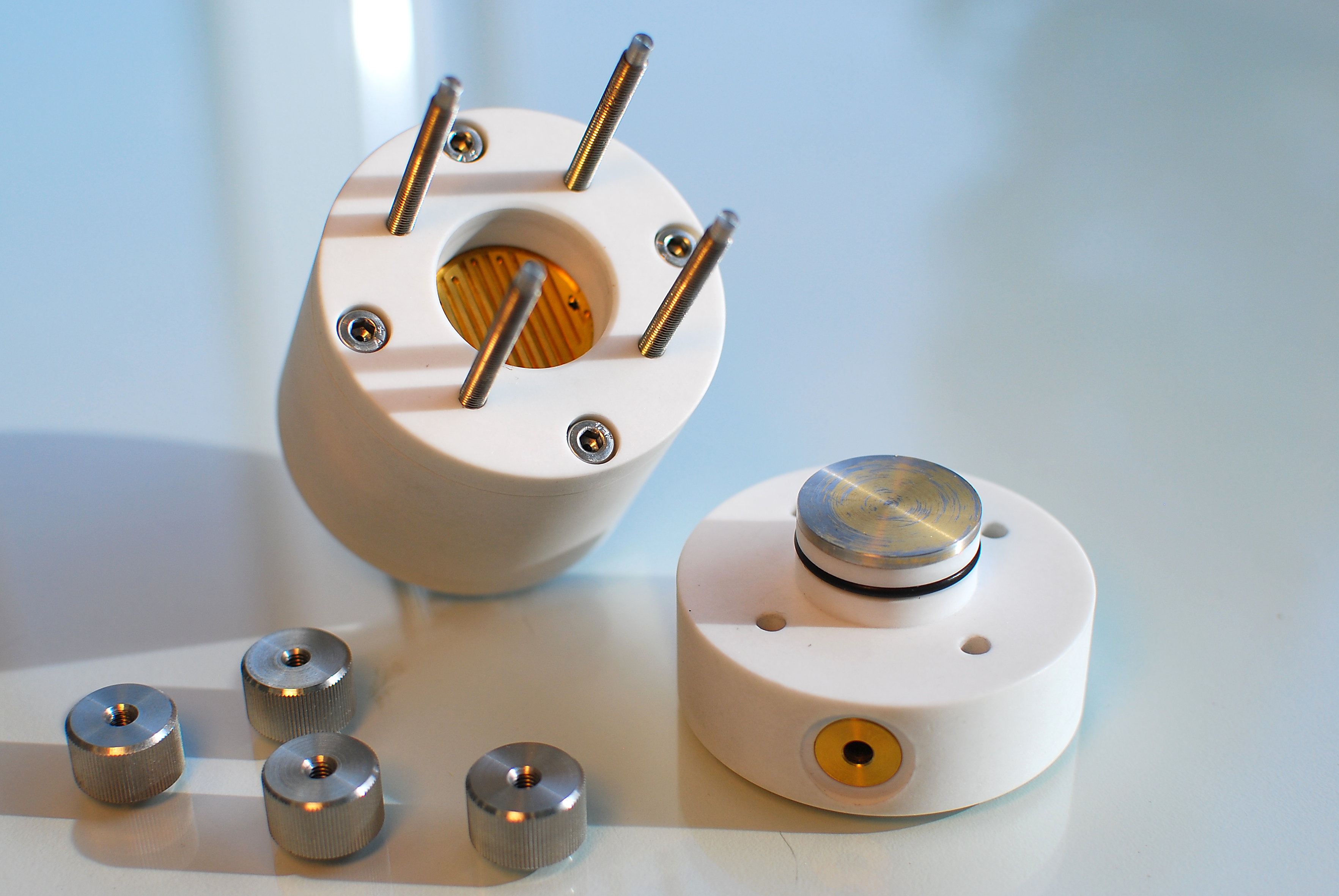
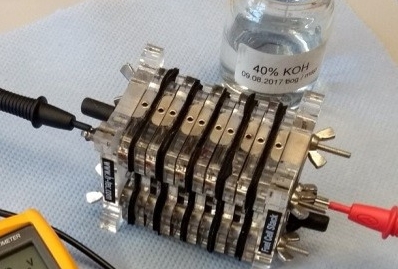
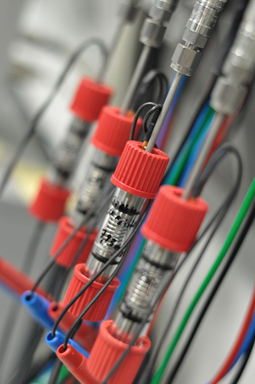
Metal-air batteries have a high energy density and constitute a potential low-cost energy storage technology. They are already commercially available as primary batteries. However, rechargeability is a major challenge and is currently the subject of research. Fraunhofer IFAM is developing rechargeable metal-air batteries. The focus is on the development of gas diffusion electrodes (GDE) with new (carbon) carrier materials and catalysts as well as novel designs with adapted porosity and wetting properties.
At Fraunhofer IFAM, various manufacturing technologies are used for metal-air batteries, such as doctor blade or roller coating of porous substrates, in-situ fabrication of mesoporous carbons, spray coating, or printing. The cell design requirements are also multi-layered, as these are "open" systems in which gaseous oxygen is the active component. Fraunhofer IFAM is developing special and also hybrid metal-air cell designs. Understanding the interaction of electrolyte and gas diffusion electrode is another main focus of our work with corresponding (in-situ) special analysis.
Alternative and high-energy energy storage
For future electromobile, stationary, and other industry-relevant applications, new battery materials and technologies are needed that represent real alternatives to the established lithium-ion battery in terms of sustainability and resource independence. Metal-air batteries have been part of energy storage research for decades and have been in development at Fraunhofer IFAM since 2009.
Metal-air technology is currently of particular interest across all industries, especially as a rechargeable variant. In addition to the potentially low-cost zinc-air system, the theoretically high-energy lithium-air system has received a great deal of attention in recent years, originally driven by the desire of the automotive industry to develop a new generation of high-energy storage systems for electromobility. Likewise, other metal-air alternatives such as calcium-air or sodium-air batteries are coming into the focus of research efforts.
Similarities and differences of the metal-air variants
There is a wide range of metal-air battery variants. The classic design of a metal-air battery consists of a metal anode, an electrolyte (solid, aqueous, organic), and a gas diffusion electrode (GDE) that provides the supply of the active oxygen component. Representative of aqueous, alkaline and organic, aprotic systems are often called the zinc-oxygen (Zn/O2) and lithium-oxygen (Li/O2) systems. Newer systems such as the Ca-oxygen (Ca/O2) system require a hybrid design, as the cathode and anode side electrolyte must be designed differently for potential stable operation. In this case, the anode compartment and cathode compartment are separated by an ion-conducting membrane (or solid-state electrolyte), which requires additional development in terms of components and cell design.
Common to all of them is the need for a gas diffusion electrode to introduce oxygen, the active component, into the cell. Mechanistically, there is a major difference in that with aqueous alkaline electrolytes the discharge products are dissolved or formed on the anode side, and in the aprotic electrolyte solid discharge products are formed which are deposited on the GDE. In the charging reaction, the conversion to the metal ions and O2 therefore takes place either electrocatalytically or homogeneously catalytically. Different heterogeneous or homogeneous catalysts or redox mediators are required to achieve efficient rechargeability.
Challenges of metal-air batteries
The known system-side challenges of rechargeable metal/air batteries are:
- dendrite-free redeposition of metal ions or cycle-stable anode
- efficient bifunctional GDE (catalysts, heterogeneous or homogeneous) for the oxygen reduction and evolution reaction (ORR/OER) during discharging and charging
- electrolyte stability towards reactive oxygen species or parasitic CO2 from the air
- cyclability
- power handling and rate capability
- open cell operation
The performance of metal-air batteries is fundamentally highly dependent on ORR and OER. Despite clearly distinguishable mechanisms in the alkaline and aprotic systems, the compatibility remains the need for catalysts to present current carrying capacity and to make the ORR and OER more efficient. This is where Fraunhofer IFAM comes in: special GDE designs and cell constructions are developed here.